The temperature of the surface of the earth is essentially determined by two things: the amount of energy coming in from the sun and the amount of energy emitted out into space by the Earth and its atmosphere. (Interestingly, that hot molten core under the earth’s crust plays very little role in the temperature of the earth at its surface.) If energy in = energy out, then the global average surface temperature of the earth will be relatively stable over the long term.
Let’s focus first on the energy-in part of Earth’s energy balance. Take a look at the diagram below. Everything in the universe, or at least everything that has a temperature above absolute zero, emits energy known as electromagnetic radiation. The wavelength that a body emits depends upon the temperature of the object itself. Shorter wavelengths carry more energy. With a blistering average temperature of about 5,800°C, the Sun emits radiation in what we call the ultraviolet, visible, and infrared parts of the electromagnetic spectrum.
Note, though, that these terms say much more about us than they do about the the sun’s light itself. There is nothing special about what we call visible light. It is just that, because it helped our forebears to survive and reproduce, we evolved sensors that could detect that part of the spectrum. Ultraviolet is just that part of the spectrum beyond violet that we cannot perceive with our eyes, and infrared is the same thing on the other side. To make it easier, I will refer to the energy emitted by the sun as solar or shortwave radiation from hereon.
Of course since the Sun is a sphere, the vast majority of the energy it emits travels in other directions and never comes near the Earth. The outer edge of the Earth’s atmosphere, however, does receive some solar radiation—an average of about 340 Watts per meter squared. Some of this incoming energy bounces off clouds and other particles in the atmosphere and never makes it to the earth. Some is absorbed by the atmosphere itself. Some of the energy that does make it to the earth is immediately reflected upwards back into the atmosphere—the so-called albedo. And of course some of this incoming solar energy is absorbed by the earth itself—warming the land and the oceans and everything upon them.
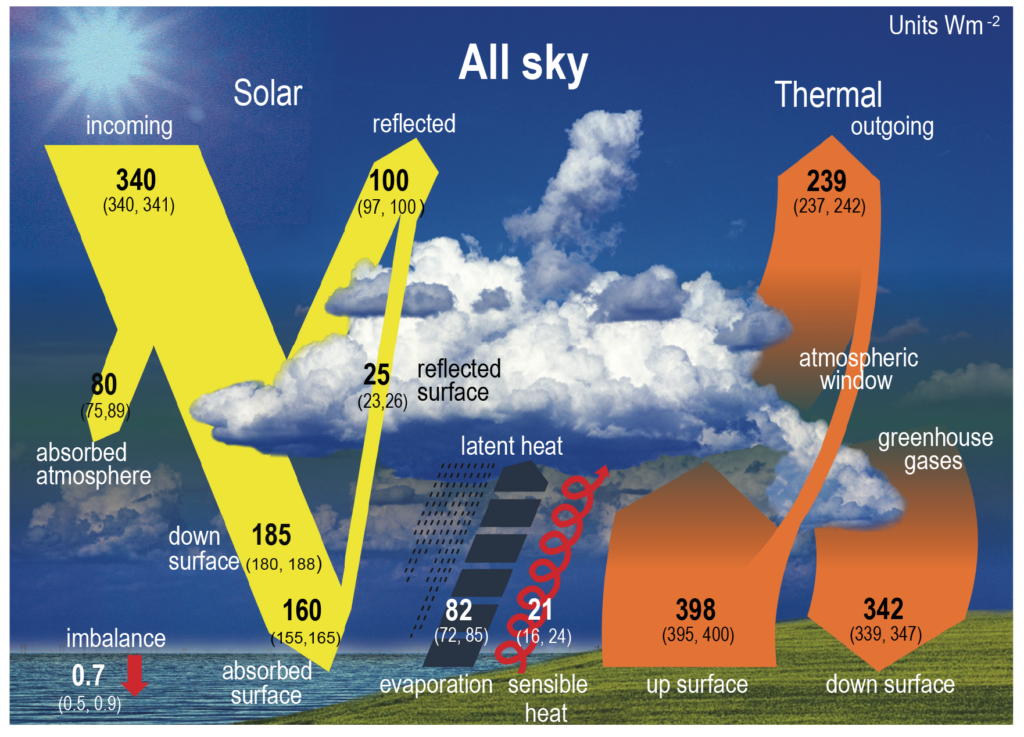
Next, let’s look at the energy out part of the earth energy budget. Just like the Sun, the Earth also emits electromagnetic radiation. However, since it is so much cooler—about 15°C—the earth emits longwave radiation in that part of the spectrum we call infrared. Some of this energy goes directly out to space. Much of it though is absorbed by gases in the atmosphere like water vapor, carbon dioxide, and methane. When these gases eventually reradiate the energy themselves, some of it is directed back at the earth, some at other gas molecules and so on. As it ricochets around between and within the earth and the atmosphere, the radiative energy is slowed or prevented from escaping into space. In other words, these gases act like a blanket or greenhouse, causing the earth to be warmer than it would otherwise be.
If the concentration of greenhouse gases in the atmosphere increases, the temperature of the Earth will also increase. A warmer Earth will emit more radiation until eventually a new equilibrium is reached.
Which finally brings us back to the concept of radiative forcing. Recall the basic equation. In a stable system: energy in = energy out. If you change either side of that equation you will cause radiative forcing. If the change causes the equilibrium temperature of the Earth to go up, it is called positive forcing. If it causes the equilibrium temperature to go down, it is negative forcing. Think of all of the radiative interactions that I described in the previous paragraphs—reflection, albedo, absorption. Launching a giant space mirror that prevented sunlight from reaching the Earth would reduce the energy in and cause negative radiative forcing. Pumping carbon dioxide into the atmosphere diminishes energy out and thereby cause positive radiative forcing.
Recent Comments